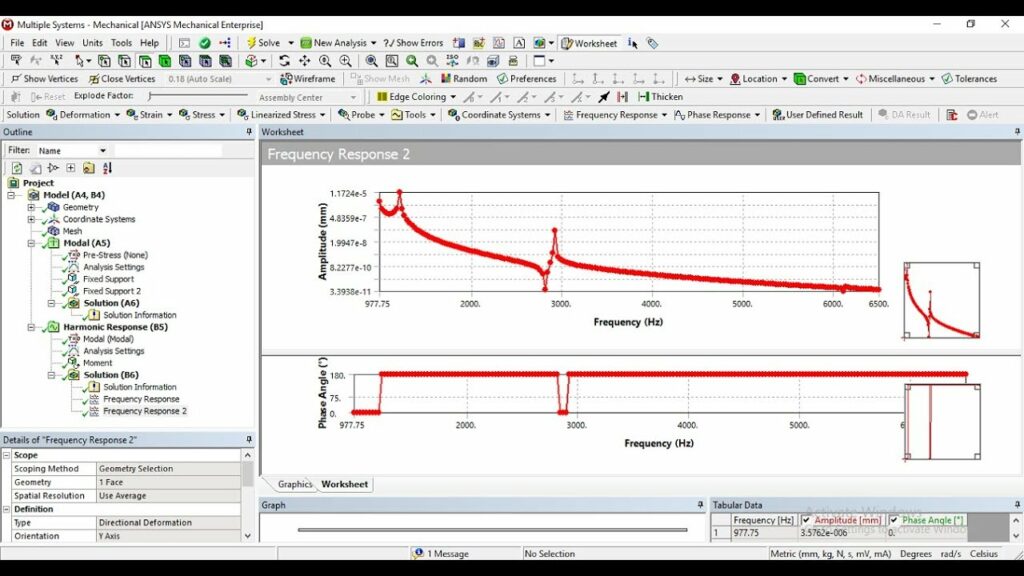
Performing Harmonic Response Analysis in ANSYS involves studying the steady-state response of structures and systems subjected to harmonic excitations or loads. This analysis is crucial in engineering design to predict the dynamic behavior of components under periodic loading conditions, such as vibrations induced by rotating machinery, wind turbines, or seismic forces. ANSYS provides robust tools and capabilities for conducting Harmonic Response Analysis (HRA), integrating advanced finite element methods with frequency domain techniques. This comprehensive guide will cover the theory, setup procedures, analysis techniques, interpretation of results, best practices, and applications of Harmonic Response Analysis in ANSYS.
Table of Contents
- Introduction to Harmonic Response Analysis in ANSYS
- Fundamentals of Harmonic Response
- Setting Up for Harmonic Response Analysis
- Performing Harmonic Response Analysis in ANSYS Workbench
- Interpreting Harmonic Response Results
- Advanced Techniques in Harmonic Response Analysis
- Best Practices for Harmonic Response Analysis
- Applications of Harmonic Response Analysis
- Case Studies and Examples
- Conclusion
1. Introduction to Harmonic Response Analysis in ANSYS
Harmonic Response Analysis (HRA) in ANSYS involves studying the response of structures or systems subjected to harmonic excitation. This type of analysis is essential for evaluating the dynamic behavior of components under steady-state periodic loading conditions, allowing engineers to assess resonant frequencies, amplitudes of vibration, stress levels, and fatigue life predictions accurately.
2. Fundamentals of Harmonic Response
- Harmonic Excitation: Periodic loading characterized by sinusoidal or harmonic functions (e.g., sinusoidal force, displacement, pressure).
- Resonance: Frequencies at which the structure’s response is amplified due to natural frequencies coinciding with excitation frequencies.
- Dynamic Stiffness: Complex-valued frequency response functions describing the system’s response to harmonic forces.
3. Setting Up for Harmonic Response Analysis
- Simulation Setup: Define the geometry, material properties, and boundary conditions of the structure in ANSYS Workbench or Mechanical.
- Loading Definition: Specify harmonic excitation parameters such as frequency, magnitude, phase angle, and direction.
- Solver Settings: Configure analysis settings, convergence criteria, and solution controls for harmonic response analysis.
4. Performing Harmonic Response Analysis in ANSYS Workbench
- Module Selection: Choose the Harmonic Response module in ANSYS Workbench.
- Frequency Range: Define the frequency range of interest for harmonic excitation.
- Frequency Sweep: Perform frequency sweeps to analyze the structure’s response across a range of excitation frequencies.
- Solver Execution: Run the simulation to obtain displacement, stress, and frequency response results.
5. Interpreting Harmonic Response Results
- Frequency Response: Visualize displacement or stress response amplitudes as a function of excitation frequency.
- Mode Shapes: Identify mode shapes and natural frequencies influencing the structure’s dynamic response.
- Resonance Identification: Determine resonant frequencies where response amplitudes are significantly amplified.
- Stress Analysis: Evaluate stress levels to ensure structural integrity under harmonic loading conditions.
6. Advanced Techniques in Harmonic Response Analysis
- Damping Effects: Incorporate damping effects to simulate realistic energy dissipation and response attenuation.
- Complex Boundary Conditions: Model complex boundary conditions such as nonlinearities or fluid-structure interactions affecting harmonic response.
- Coupled Physics Analysis: Perform coupled harmonic response analysis involving multiple physics domains (e.g., structural-acoustic coupling).
7. Best Practices for Harmonic Response Analysis
- Frequency Resolution: Ensure adequate frequency resolution to capture critical resonant frequencies and response peaks.
- Convergence Testing: Conduct convergence studies to verify solution accuracy and stability across different frequency ranges.
- Model Validation: Validate harmonic response predictions through experimental testing or comparison with analytical solutions.
- Post-Processing Techniques: Use advanced post-processing techniques to extract and analyze critical response data effectively.
8. Applications of Harmonic Response Analysis
Harmonic Response Analysis in ANSYS finds applications across various industries and engineering disciplines:
- Mechanical Engineering: Assess vibrations in machinery, automotive components, and rotating structures.
- Aerospace Engineering: Evaluate structural dynamics and resonance effects in aircraft and spacecraft components.
- Civil Engineering: Analyze seismic vibrations and wind-induced vibrations in buildings and bridges.
- Consumer Electronics: Predict and mitigate vibrations in electronic devices and consumer products.
9. Case Studies and Examples
- Automotive Suspension Analysis: Optimizing suspension components for reduced vibration and improved ride comfort.
- Wind Turbine Blade Dynamics: Evaluating structural responses to harmonic wind loads for enhanced durability.
- Medical Device Design: Assessing vibrations and resonances in medical equipment for reliability and patient safety.
10. Conclusion
Harmonic Response Analysis in ANSYS is a powerful tool for predicting and understanding the dynamic behavior of structures subjected to periodic loading conditions. By leveraging ANSYS’s advanced simulation capabilities, engineers can optimize designs, minimize risks of resonance-induced failures, and ensure structural integrity across diverse engineering applications. Mastering harmonic response analysis techniques empowers engineers to innovate, improve product performance, and deliver robust solutions that meet stringent design requirements and exceed customer expectations in dynamic environments.